The latest publication of the top issue of
Guide
Covalent organic frameworks (COFs) have emerged as a promising platform for nanomedicine, but developing multifunctional COF nanoplatforms remains challenging due to the lack of effective COF modification strategies. Herein, a nanozyme bridging (NZB) strategy for COF functionalization is proposed. Platinum nanoparticles (Pt NPs) as catalase mimics were grown in situ on the surface of COF NPs without reducing their drug-loading capacity (CP), and thiol-terminated aptamers were further densely decorated onto CP NPs via stable Pt-S bonds (CPA). Pt nanozyme engineering and aptamer functionalization endow the nanoplatform with excellent photothermal conversion, tumor targeting, and catalase-like catalytic properties. Using the clinically approved photosensitizer indocyanine green (ICG) as a model drug, a nanosystem for tumor-targeted self-reinforcing therapy (ICPA) was fabricated. ICPA can efficiently accumulate into tumor tissues to alleviate the hypoxic microenvironment by decomposing overexpressed H2O2 and generating O2. Under single-wavelength near-infrared light irradiation, the catalase-like catalytic and singlet oxygen generation activities of ICPA were significantly enhanced, resulting in a favorable photocatalytic therapeutic effect on malignant cells and tumor-bearing mice in a self-reinforcing manner.
Graphic reference
Figure 1. Synthesis of ICPA and its application to reverse tumor hypoxia, induce tumor thermal damage, and oxidative stress in a self-reinforcing manner under single-wavelength near-infrared light irradiation
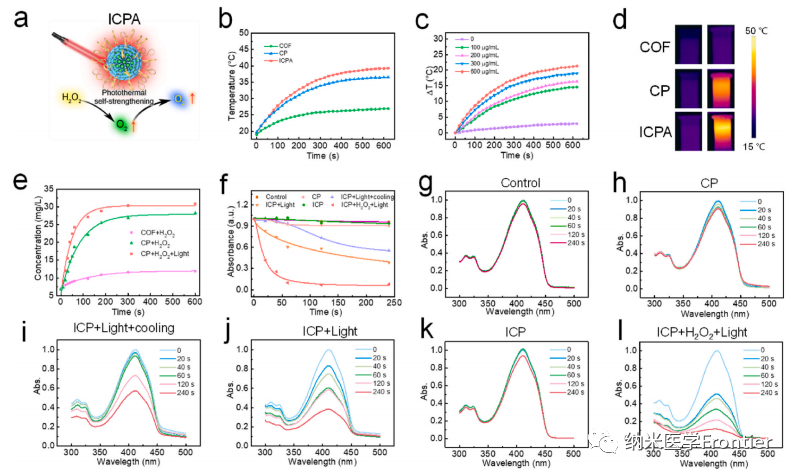
Fig. 3. (a) Schematic diagram of ICPA with self-enhancement mode under single-wavelength near-infrared radiation. (b) Photothermal curves and (d) photothermal images of various nanoparticles (600 μg/mL) under 808 nm light irradiation (1 W/cm2). (c) Photothermal curves of different concentrations of ICPA under 808 nm laser (1 W/cm2). (e) Oxygen production capacity of different materials with different treatments measured by dissolved oxygen meter. (f) DPBF absorption decay rate caused by different nanoparticles. (g)–(l) UV-vis spectra of DPBF after different treatments.
Figure 5. (a) In vivo fluorescence imaging of 4T1 tumor-bearing mice injected with ICPA or ICP at different time points and (c) quantification of the fluorescence intensity of the tumor area in (a). (b) Thermal images of mice at different time points after intravenous injection of ICPA and (d) temperature quantification of (b). (*p < 0.05, **p < 0.01, ***p < 0.001).
Figure 6. (a) Schematic diagram of animal experiments. (b) Body weight change curves of mice receiving different treatments within 12 days. (c) Relative tumor volumes of different groups. (d–i) Tumor volumes in mice with different treatments. (j) Photographs of tumor-bearing mice after different treatments. (k) H&E staining of tumor sections after different treatments. (I, PBS; II, light; III, ICPA; IV, CPA + light; V, ICPA + light + cooling; VI, ICPA + light).
Summarize:
The Pt-COF-Apt nanoplatform has good water solubility, stability, biocompatibility and tumor targeting ability. In in vitro experiments, the Pt-COF-Apt nanoplatform can effectively recognize and kill tumor cells, showing a highly efficient photocatalytic therapeutic effect. In vivo experiments, the Pt-COF-Apt nanoplatform can effectively accumulate at the tumor site to generate a large number of reactive oxygen species, thereby inhibiting tumor growth and metastasis. This experiment demonstrates the Pt-COF-Apt nanoplatform as a novel photosensitizer, providing a new strategy for tumor-targeted self-enhanced photocatalytic therapy. Photocatalytic therapy is a method that uses photosensitizers to generate reactive oxygen species under light, thereby killing tumor cells. This method has the advantages of noninvasiveness, high efficiency, and low toxicity, but there are also some problems, such as the stability, selectivity, and photocatalytic efficiency of the photosensitizer.
Innovation:
1. This study prepared a new type of nanomaterial ICP, which has excellent photothermal conversion ability and antitumor drug loading ability, and can be used for tumor-targeted photothermal therapy. A platinum nanozyme-bridged covalent organic framework (Pt-COF) was used as a photosensitizer to combine with an aptamer (Apt) to form a Pt-COF-Apt nanoplatform.
2. Evaluated the photothermal conversion ability and anti-tumor drug loading capacity of ICP, and found that it can generate higher temperature under near-infrared light irradiation, and can effectively load anti-tumor drugs. 3. Evaluated the anti-tumor effect of ICP and found that it can effectively inhibit tumor growth both in vitro and in vivo.
+86-18915413828(WhatsApp&WeChat)
Previous: New breakthroughs in c
Next: 《Nature》Biomimetic pro