Angew: New member! Mechanically discolored perovskite nanosheet structural color hydrogel
1. Article overview
The structural colors of natural opal, beetles, butterflies, abalone shells, etc. are produced by the interference or diffraction of light by the submicron microstructure of the material, unlike dyes and pigments, which are caused by electronic transitions caused by light absorption. Because structural color materials have fascinating properties, such as high durability and peculiar rainbow colors, they are expected to be widely used in the fields of painting, anti-counterfeiting and decoration. Inspired by nature, researchers have been working on making structural color materials, such as the assembly of spherical particles, cholesteric liquid crystals, and surfactant micelles. In addition, the coupling of structural color characteristics with other functions is further optimistic, and new materials that are beyond the scope of natural systems may be produced. Stimulus-responsive structural color materials are suitable for sensors, smart devices, smart displays, and information storage. Although cholesteric liquid crystals have been referred to as classic temperature-responsive structural colorants, it has recently been reported that soft elastomers or gels with structural colors are mechanically chromogenic materials.The research team of Professor Nobuyoshi Miyamoto of Fukuoka Institute of Technology in Japan reported in this article a functional perovskite nanosheet as a new member of the structural color material; the researchers combined this structural color perovskite nanosheet into the structure by in-situ photopolymerization In the poly(N-isopropylacrylamide) (PNIPAm) network, a mechanically discolored structural color hydrogel film with fast response, high sensitivity and high mechanical toughness has been successfully fabricated. Due to the spontaneous orientation of liquid crystal (LC) nanosheets at the liquid-glass interface, no special equipment is required to obtain uniformly aligned monodomains of nanosheets. The Dion-Jacobson type layered perovskite oxide is selected here, and its general composition formula is expressed as M [An-1BnO3n+1] (M = K, Na, H; A = Ca, Ba, K, Sr; B = Nb, Ti). Due to the highly designable chemical composition, structure and function (such as luminescence characteristics, photocatalytic activity and solid acid catalytic activity), this strategy lays the foundation for the development of multifunctional structural color materials in the future.
Two, graphic guide
The bright structural color of perovskite nanosheet colloid can be adjusted by changing the concentration of nanosheets. The 3wt% (Figure 1d) and 2wt% (Figure 1c) nanosheet colloids showed a structural color with pearl luster, while the 1 wt% (Figure 1b) colloid was colorless. In the UV-visible reflectance spectrum, the 3 wt% colloid (Figure 1d) shows a maximum reflectance wavelength max of 469 nm. In addition, a small peak is also observed in the ultraviolet region (3 wt%) near 238 nm, that is, half of max. The strong absorption below 360 nm is attributable to the band gap absorption of the semiconductor perovskite nanosheets, which has been confirmed by the following observations: Band gap absorption is also observed in unstructured dilute colloids (0.1 wt%) ( Figure 1a). As the concentration of nanoplatelets decreases, max shifts to longer wavelengths: 600 nm and 760 nm at 2 wt% and 1 wt%, respectively.
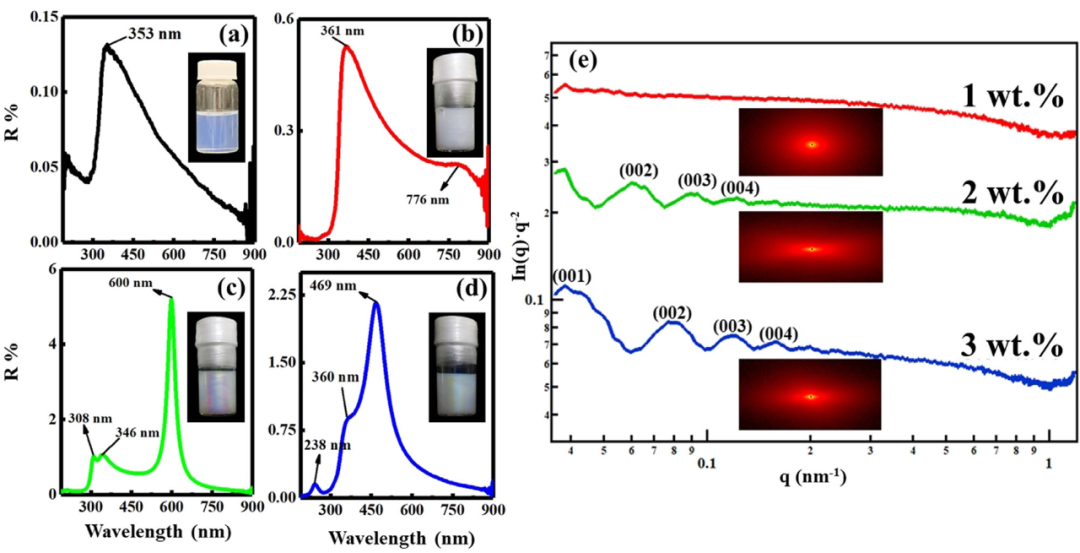
Figure 1. The structure of the liquid crystal perovskite nanosheets. The characteristics of the colored colloidal sol.
a-d) The ultraviolet-visible reflectance spectra and photographs of the colloidal nanosheets with a concentration of a) 0.1 wt%, b) 1 wt%, c) 2 wt%, and d) 3 wt%.
e) Small-angle X-ray scattering curves and 2D patterns of colloids with 1 wt%, 2 wt%, and 3 wt% nanosheets.
A) UV/Vis spectra of samples in each step of gel synthesis.
B) Polarized optical microscope images of the cross-section of the gel observed with crossed polarizers 08 and 458 set on the surface of the gel.
C) The SAXS profile of the gel film observed from the cross section (red line) and the surface (black line).
D) Illustration of the surface, slice surface and cross-section of the gel used for SEM and SAXS observation.
E-I) SEM image of the dried gel: E), F) the surface of the composite gel and the surface of the slice; G), H) the cross section of the composite gel; I) the SEM image of the surface of the pure PNIPAm gel.
A) Compressive stress-strain curves of hydrogels with different nanosheet concentrations of 0, 1, 2 and 3 wt%.
B) The relationship between the compressive strain and the max of the gel. Illustration: Photo of the gel film attached to the glass plate; use tweezers to push one end of the glass plate so that the gradient color appears due to the pressure gradient (C) with different nanosheet concentrations of 0, 1, 2 and 3 wt% water The tensile stress-strain (ss) curve of the gel.
D) Photograph of a gel with 3wt% nanoplatelets during the tensile test; the photo was taken at the point indicated in the s-s curve of (C).
E) A photo taken with a high-speed camera when the gel is hit by a heavy object.
F) Demonstrate that the letter "FIT" can be displayed reversibly by pushing the structural color glue between the glass plate and the stamp made of silicon rubber sheet.
+86-18915694570(WhatsApp&WeChat)
Previous: Southwest Jiaotong Uni